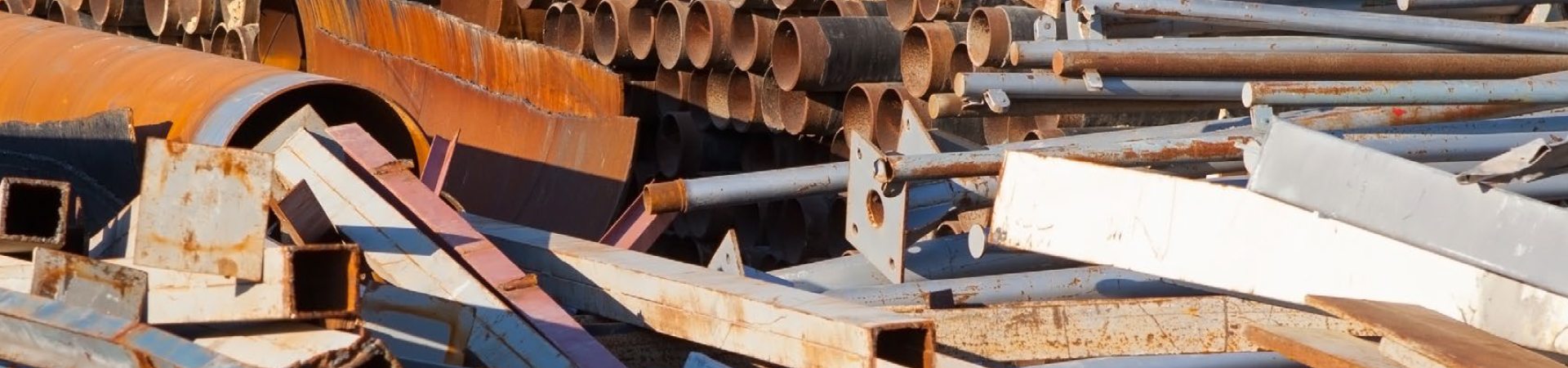
Sustainability and Steel : Frequently Asked Questions
Sustainability Frequently Asked Questions
The British Constructional Steelwork Association and Steel for Life have produced a series of 11 frequently asked questions (FAQs), with the support of the Steel Construction Institute (SCI), that aim to tackle several specifier questions focussed on steel, sustainability, and climate change.
Readers can view the shortened answers or a longer more details answer to each FAQ’s.
Should I specify a high percentage of recycled material?
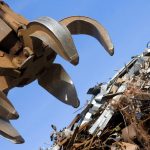
Recycling is good for the planet because it reduces waste and conserves (or substitutes) primary resources, furthermore recycling generally saves energy and reduces carbon emissions.
Specifying a high recycled content is a good driver for products where the aim is to encourage and establish a market for recycled materials that are otherwise limited, uneconomic or immature. This is not the case for steel however, which is already highly recycled (> 95% in the case of structural steel). Specifying steel with a high recycled content drives no positive climate impact at a global level, can have adverse consequences within the broader sustainability context and create market distortions.
It should be remembered that both major steel production routes use scrap. The primary or Blast Furnace-Basic Oxygen Furnace (BF-BOF) route up to 30% recycled content and the secondary Electric Arc Furnace (EAF) route 100%.
Specifying a high recycled content can be beneficial for some products however that is not the case for metals that are already highly recycled. Indeed, specifying steel with a high recycled content can have adverse consequences within the broader sustainability context.
In our daily lives we are all encouraged to recycle more. Recycling is good for the planet because it reduces waste and conserves (or substitutes) primary resources, furthermore recycling generally saves energy and carbon emissions.
But how should we measure and incentivise recycling? The answer to this is more complicated than the simple ‘recycling is good’ and ‘recycle more’ messages and needs to be understood by specifiers of construction products if unsustainable and unintended consequences are to be avoided.
There are two main ways of measuring recycling, recycled content and end-of-life recycling rate:
Recycled content is defined in ISO 14021 [1] and is a measure of how much recycled content (pre- and post-consumer) is used in the production of a new product
End-of-life recycling rate is a measure that compares the actual amount of materials recycled with the amount of metals theoretically available at the end of the life of a product. In the context of construction, this is the proportion of demolition arisings that are recycled
Specifying a high recycled content is a good driver for products where the aim is to encourage and establish a market for recycled materials that are otherwise limited, uneconomic or immature.
For example, the recycled content approach may be a useful metric/driver for a material that would otherwise be incinerated or landfilled as waste. Specifying a recycled content for products incorporating these materials, can divert them from incineration and landfill to recycling and reuse. Importantly, this is not the case for metals - as discussed below, metal recycling is economical and the metals recycling market is extensive and mature.
The application of the recycled content approach may create market distortions and environmental inefficiencies. If a designer specifies high recycled content in a well-meaning effort to reduce environmental impacts, it may stimulate the market to direct recycled feedstock towards designated products and away from production where recycling is most economical. For metals, where there is a limited supply of recycled feedstocks, market stimulation is ineffective and may result in inefficient processing and unnecessary transportation. See the declaration on recycling principles by the metals industry [2].
1.1 Global steel production
Global consumption of steel products continues to rise, mainly as a consequence of industrialisation in developing economies and particularly, in recent years, growth in China. For example, the World average per capita consumption of crude steel in 2019 was 229 kg. By comparison, the EU-28 average consumption is 310 kg/capita, UK consumption was 151 kg/capita and average consumption in Africa was just 28 kg/capita [3].
Fig. 1. Growth in global steel production 1950-2019, worldsteel [3]
Fig. 2. Anticipated global demand for steel to 2050 [4]
Anticipated global demand for steel to 2050 Fig. 2. Anticipated global demand for steel to 2050 [4] Global demand for new steel exceeds the supply of scrap steel by a factor of around two and therefore, to meet this increasing demand, new steel has to be produced from primary sources by the BF/BOS production route. Supplies of ferrous scrap are finite and exclusively specifying 100% recycled steel, will result in an increased demand for scrap that is not only unsustainable but may also drive up scrap prices.
Within the global context, there is no point specifying 100% recycled steel (in preference to BF/BOS steel) as all this will mean is that BF/BOS steel will be required to substitute EAF production to meet the excess global demand elsewhere. In addition, specifying imported 100% recycled steel in the UK, adds further transportation impacts.
The corollary of this is that on a global scale and while global demand for new steel exceeds the global supply of scrap, it is the end-of-life recycling rates achieved (not the recycled content) that is important for sustainable development.
This is not just the view of the metals industries. The following are extracts from other, independent sources.
WRAP undertook a number of studies promoting the use and specification of recycled content for construction products. However, for metals, WRAP concluded [5]: For recycled content within the metals industry, where recycling is already optimised (where over 90% of waste arising is recycled or reused), an industry average for each product type, e.g. aluminium sheet or steel sections, should be declared, rather than on a site-by-site or company-by-company basis. If, for example, steel sections available globally to the UK market have 60% recycled content on average, then the steel industry could all use that figure as the recycled content of sections.
In her article [6] Kirsten Henson states: Rather than insisting on high recycled content in structural steel, which ultimately drives no positive climate impact on a global scale (due to the fact that the majority of steel is already captured at end-of-life and recycled) and potentially contributes to the further decline of the UK steel industry, architects and building owners must seek innovative ways of re-using existing structures and engineers must use ingenuity to deliver the most materially efficient design solutions.
The ICE database V2.0 [7] includes an excellent, detailed treatise on how to account for the recycling of metals in embodied carbon assessments. The ICE authors share the views of the metals industry about market possible distortions that can result from specifying a high recycled content for steel. Consequently, the ICE database, which is ‘cradle-to-gate’ (Modules A1 – A3) only, recommends the implementation of an effective average recycled content for steel products and states: To implement this method effectively the same recycled content must be modelled for all different forms of (carbon) steel (e.g. rebar, sheet or section). Technological and economic factors often dictate where scrap materials are directed. The fact that a certain type of steel is almost exclusively manufactured from primary feedstock (iron ore) or secondary feedstock (scrap steel) becomes irrelevant. For example, steel rebar is often manufactured in an electric arc furnace (EAF) and therefore often has a recycled content of 100%. Conversely steel sheet is typically derived from a primary production route. An increase in the demand and production of steel rebar will not result in an increase in the global average recycled content for worldwide steel. Likewise, an increase in the demand and production of steel sheet will not reduce the global average recycled content. Scrap steel remains a valuable commodity and will be consumed regardless of the demand for certain types of steel. Consequently, changes in the demand of certain forms of steel (rebar, sheet or section) have no capacity to increase the global average recycled content. The consequences of this are that although rebar is normally made from recycled steel and sheet is typically made from virgin steel both materials are given an ‘effective market average recycled content’ within the ICE database.
Why is European steel lower carbon than UK steel?
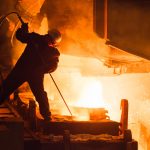
The lower average carbon footprint of European steel production is simply a function of the steel production split within Europe compared to that in the UK.
Steel is predominantly manufactured by either of two process routes, namely the primary or basic oxygen steel-making route (BF-BOF) and the secondary, electric arc furnace (EAF) route.
In Europe the production split (for all steel) is around 60:40 (BF-BOF:EAF). In the UK the production split is currently 80:20 (BF-BOF:EAF).
Typically, the EAF steel-making process has a carbon footprint around 20% that of BF-BOF steelmaking. Therefore, the European and UK average carbon footprint values for steel reflect these different production splits.
As a globally traded product, a UK average production mix for steel sections is meaningless. Instead, and where the steel supplier is unknown, for example at the early design stage, the BCSA recommends the following average UK emissions factors for embodied carbon assessments of structural steelwork:
- For Modules [A1-A3], 1.74 tonne CO2e per tonne of sections.
- For Module [D], - 0.93 tonne CO2e per tonne of sections.
Although on average this may be true, it is not the case on an individual company or individual steel mill basis. This is one of the downsides of using average production values for steel making carbon emissions. The lower average carbon footprint of European steel production is simply a function of the production split within Europe compared to that in the UK, as explained below.
Steel is predominantly (> 99% globally) manufactured by either of two process routes, namely the basic oxygen steel-making route (BF-BOF) and the electric arc furnace (EAF) route. Globally, in 2019, the production split was circa 70% BF-BOF and 30% EAF [3].
In basic oxygen steelmaking, molten iron is produced from iron ore, using a Blast Furnace (BF), and then refined in a BOF converter to which scrap steel is also added (up to 30%). The actual proportion of scrap is dependent upon a number of factors including the availability and price of scrap and the specification or quality of the steel being produced. In addition to saving iron ore, the scrap is an important part of the steel-making process, i.e. it is used to regulate the temperature of the steel in the furnace. EAF production is primarily a scrap-recycling route, which involves melting 100% steel scrap to which small amounts of fluxes and reducing agents are added.
In Europe, both steel-making routes are used, the production split (for all steel) being around 60:40 (BF-BOF:EAF) in 2019. In the UK the production split is currently 80:20 (BF-BOF:EAF) [3].
Typically, the EAF steel-making process has a carbon footprint around 20% that of BF-BOF steelmaking. Therefore, the European and UK average carbon footprint values for steel reflect these production splits.
As a globally traded product, a UK average production mix for steel sections is meaningless. Instead, and where the steel supplier is unknown, for example at the early design stage, the BCSA recommends the following average UK emissions factors for embodied carbon assessments of structural steelwork:
- For Modules [A1-A3], 1.74 tonne CO2e per tonne of sections
- For Module [D], - 0.93 tonne CO2e per tonne of sections
Whereas there are historical and other reasons why the production split varies between different countries and regions, globally the ability to make steel via the EAF route is limited by the availability of steel scrap. This explains why, despite unrivalled end-of-life recovery rates for steel products, see [8], it is not currently possible for all designers to specify EAF steel. This will change however as the stock of steel in buildings and infrastructure in developing countries grows and stabilises. At this point, a steady state will exist in which demand for new steel can be met entirely through the supply of scrap and no further BF-BOF or primary steel production is required, see Box 1 for further information. The challenge for the steel industry and wider society, is how we transition to this sustainable ‘steady state’ against the backdrop of addressing the current climate emergency.
Although there is some variation in BF-BOF carbon emissions between steel companies, this variation is small and is mainly a function of the proportion of scrap used in the process. BF-BOF production is highly efficient, and the average energy intensity of steel making is now within 10% of best practice and best practice is just double the unattainable absolute theoretical limit. No other industry operates so close to its theoretical performance limit [4]. BF-BOF emissions for structural sections and plates are around 2.4 tCO2e per tonne.
EAF carbon emissions vary depending primarily on the electricity grid mix in the country or region of production. EAF emissions for structural steel sections in Europe is currently around 0.5 tCO2e per tonne. Policies to decarbonise the electricity grid should reduce these emissions further.
Transition of the global steel industry from primary to secondary steelmaking
Fig 2 Anticipated global demand for steel to 2050 (4)
Figure 2 shows a prediction of global steel demand to 2050. Although it is difficult to predict the future with certainty, experience shows that as countries develop their per capita requirement for steel levels off at a stock of around 12 tonnes of steel per person.
Fig 3 and 4 The stock of steel (tonnes/person) relative to GDP/person
Figure 3 shows the rise in steel stock in five developed countries over time as GDP increases [4]. Demand for steel after this ‘saturation point’ is for replacement, i.e. recycling, rather than expansion through BF-BOF production from iron ore.
Combining this ‘saturation value’ with the predicted life expectancies of steel products, it is possible to predict future global demand and, assuming that most future scrap arisings are recycled, how the global split of BF-BOF and EAF production and associated carbon emissions, will evolve over time. Figure 4 shows this.
Global steel demand will eventually reach a limit when steel stocks stabilise and the requirement for primary production reduces to (nearly) zero, as future requirements are met entirely through recycling.
Further grid decarbonation will enable us to have a truly sustainable, zero carbon, global steel industry. The challenge the steel industry is now tackling is how to reduce BF-BOF carbon emissions while we still need steelmaking from iron ore and we transition to steel-making based solely on recycling scrap and reuse.
Should I rationalise my design?
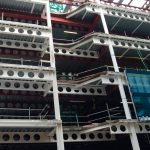
Rationalisation of steelwork design is common practice on most projects in the UK. The main drivers for this are constraints on design fees and time and efficiencies in procurement, fabrication and erection of the steelwork.
Reviews of steelwork designs have shown that some material efficiency savings are achievable by not rationalising designs but these studies also conclude that the main cause of over-specified steelwork is conservative engineering assumptions to retain a degree of flexibility or reserve in structural capacity.
Rationalisation of steelwork design is current, common practice on most projects in the UK. The main drivers for this are constraints on design fees and time and efficiencies in procurement and fabrication and erection of the steelwork.
There have been several recent studies looking specifically at the material efficiency of steel structures, see, for example, references [9-12]. These studies suggest that over-specification of sections can lead to an additional 30-40% of steel used within the structure and, therefore, to additional embodied carbon within the structure. While bespoke designs may contribute to reducing the upfront embodied carbon, there are good reasons to rationalise designs:
- from a safety point of view, less variability in member sizes reduces the possibility of human error during erection
- from an execution standpoint, the increase in the speed of construction
- from Other studies have investigated other factors including over-specification of loads and less conservative SLS criteria where SLS governs. While these aspects may help reduce embodied carbon within the structure, they are unrelated to rationalisation of the steelwork design.a sustainability or circular economy perspective, rationalised designs enable greater flexibility and adaptability of the building and enhance the reusability of the structural elements
It is noted that [10] concluded that rationalisation or repetition within the structure could not explain the over-specified mass of the steel frame rather it is the designers decision to limit the utilisation ratio to 0.8 that was the main cause. In addition, there is unused mass in cores, trimmers, ties, etc. and in edge beams which are under-utilised but are necessary for stability. Figure 5 (from [9]) shows the utilisation as a function of beam type from an analysis of 30 buildings.
Other studies have investigated other factors including over-specification of loads and less conservative SLS criteria where SLS governs. While these aspects may help reduce embodied carbon within the structure, they are unrelated to rationalisation of the steelwork design.
Fig. 5. Utilisation ratio as a function of beam type [10]
How strong is S355 really?
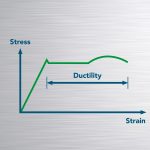
S355 steel is a European standard structural steel grade which has a minimum yield strength of ReH,min = 355 N/mm2 for the smallest thickness range covered by the product standard. This is the nominal value of the yield stress, as opposed to the actual measured yield strength (which is usually higher). Steel structures designed to Eurocode 3 adopt nominal values of the steel properties as characteristic values in design calculations.
The new Annex E of prEN 1993-1-1:202X gives statistical data of properties for materials currently available on the European market, satisfying the relevant European product standards. For steel grade S355, the mean value Xm is 1.20ReH,min = 426 N/mm2 with a coefficient of variation of 5%. The values currently adopted for partial factors ?M are calibrated on this statistical data, considering a reliability index of 3.8 for a reference period of 50 years. This means that for design purposes, the nominal value cannot simply be substituted by, for example, a mean value without proper re-calibration of the partial factors in order to satisfy the reliability requirements from Eurocode 0.Rationalisation of steelwork design is common practice on most projects in the UK. The main drivers for this are constraints on design fees and time and efficiencies in procurement, fabrication and erection of the steelwork.
Reviews of steelwork designs have shown that some material efficiency savings are achievable by not rationalising designs but these studies also conclude that the main cause of over-specified steelwork is conservative engineering assumptions to retain a degree of flexibility or reserve in structural capacity.
S355 steel is a European standard structural steel grade which has a minimum yield strength of ReH,min = 355 N/mm2 for the smallest thickness range covered by the relevant standard – i.e. BS EN 10025. This is the nominal value of the yield stress, as opposed to the actual measured yield strength.
Steel structures designed to Eurocode 3 (British Standard) adopt nominal values of the steel properties as characteristic values in design calculations. Eurocode 0 defines characteristic value as “the value of a material property having a prescribed probability of not being attained in a hypothetical unlimited test series. This value generally corresponds to a specified fractile of the assumed statistical distribution of the particular property of the material.” Clause 4.2(3) of BS EN 1990:2002+A1:2005 further states that:
- where a low value of material or product property is unfavourable, the characteristic value should be defined as the 5% fractile value
- where a high value of material or product property is unfavourable, the characteristic value should be defined as the 95% fractile value
Typically, the actual yield strength is higher than the nominal value. The new Annex E of prEN 1993-1-1:202X gives statistical data of properties for materials currently available on the European market, satisfying the relevant European product standards. For steel grade S355, the mean value Xm is 1.20ReH,min = 426 N/mm2 with a coefficient of variation of 5%. Annex E also gives an upper reference value, X5%, and a lower reference value, X0.12%. They refer to non-exceedance probabilities of 5% and 0.12%, respectively. For S355:
- X5% = 1.11ReH,min = 394 N/mm2
- X0.12% = 1.03ReH,min = 366 N/mm2
It should be stressed that the values currently adopted for partial factors ?M are calibrated on this statistical data, considering a reliability index taken equal to 3.8 for a reference period of 50 years.
This means that for design purposes, the nominal value cannot simply be substituted by, for example, a mean value without proper re-calibration of the partial factors in order to satisfy the reliability requirements from Eurocode 0.
How does the circular economy help today’s emissions?
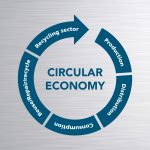
There are many different facets to the circular economy including material efficiency, reuse and recycling. Steel has excellent circular economy credentials both as a material which is strong, durable, versatile and recyclable and, as a structural framing system, which is lightweight, flexible, adaptable and reusable.
While the focus of the circular economy in construction is on how to transition from a linear to a circular model, because of steel’s excellent recycling credentials steel is already circular with very little ‘leakage’ out of the system. Recovery rates for structural steel, for reuse and recycling, are over 95%. Globally, these high recovery and recycling rates translate into savings of around 950 mtCO2 per year.
A circular economy is based on the principles of designing out waste and pollution, keeping products and materials in use, and regenerating natural systems. Figure 6, developed by the Ellen McArthur foundation, is one of the best accepted representations of circular economy concepts. The left hand (green) half of the figure represents biological cycles such as timber and the right-hand side (blue) represents technical materials, including, in the context of construction, concrete, metals, plastics, etc.
There are two fundamental concepts to highlight within the figure:
- Leakage out of the circular system, to energy recovery or landfill, should be avoided wherever possible
- Within the concentric circles, the smaller the diameter the more efficient the process
Steel has excellent circular economy credentials both as a material which is strong, durable, versatile and recyclable and, as a structural framing system, which is lightweight, flexible, adaptable and reusable.
While the focus of the circular economy in construction is on how to transition from a linear to a circular model, because of steel’s excellent recycling credentials [8] steel is already circular with very little ‘leakage’ out of the system. Recovery rates for structural steel, for reuse and recycling, are over 95% and, with steel recycling emitting only 20% of the carbon emissions compared to making steel from iron ore, steel is already helping significantly to reduce carbon emissions.
But the steel sector is not content with just recycling and is looking to improve its circular credentials further by encouraging greater steel reuse and designing to facilitate future deconstruction and reuse. Steel structures and structural steel elements are inherently demountable and reusable. While there are currently logistical and supply chain barriers to more mainstream reuse, reuse is technically viable, saves resources and carbon emissions and can save money.
To help facilitate structural steel reuse, SCI has recently published guidance documents:
- SCI P427 Structural steel reuse: a protocol for reusing existing structural steel
- SCI P428 Guidance on demountable composite construction systems for UK practice
Fig. 6. Representation of the circular economy – Ellen MacArthur Foundation
Why are some steel construction products preferentially produced by different production routes?
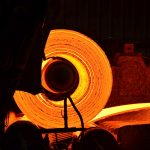
All steel construction products can be produced using either of the two principle steelmaking routes, i.e. primary Blast Furnace-Basic Oxygen Furnace (BF-BOF) or the secondary, Electric Arc Furnace (EAF) route. However, for various technical and economic reasons, some products are preferentially produced by one or other of these routes.
The central issue relates to the quality control of the raw materials input to the steelmaking process and the required quality of the finished product. In BF-BOF steelmaking, the primary raw material input is iron ore and controlling the quality of the iron ore is relatively easy and therefore high and consistent quality steel can be made cost effectively via this route. The quality is particularly important for producing flat, relatively thin products like plate.
In EAF steelmaking, the primary input is scrap steel and the quality of the steels produced are dictated by the quality and blend of the scrap. Scrap steel is usually contaminated with tramp elements and some of these elements remain in the finished products which can affect its quality. Therefore, if high quality control, i.e. sorting and blending of scrap is done, steels of high quality, such as stainless or engineering steel, can be produced. On the other hand, if the quality control is low then relatively low quality steels can be cost produced via EAF cost effectively.
For the above reasons, structural steel plate used in the UK is produced via the BF-BOF. Hot-rolled sections are produced, equally efficiently, via either production route.
Steel is used in numerous construction products, however the main generic groups are:
- Rolled sections used as structural members and for piling
- Rebar and mesh used for reinforcing concrete
- Steel plate used to fabricate larger structural elements
- Hollow or closed sections used as structural members
- Light gauge flat steel generally galvanized, used in many construction applications including floor decking, cladding and studs for light steel framing
All of these products can be produced via either of the two principle steelmaking routes, i.e. the primary Blast Furnace-Basic Oxygen Furnace route (BF-BOF) or the secondary, Electric Arc Furnace (EAF) route. However, for various technical and economic reasons, some products are preferentially produced by one or other of these routes. In some countries, geographical and historical factors also influence the preferred steelmaking route for some products. The availability and cost of scrap is also a factor.
The central issue relates to the quality control of the raw materials input to the steelmaking process and the required quality of the finished product. In BF-BOF steelmaking, the primary raw material input is iron ore and controlling the quality of the iron ore is relatively easy and therefore high and consistent quality steel can be made cost effectively, via this route.
In EAF steelmaking, the primary input is scrap steel and the quality of the steels produced are dictated by the quality and blend of the scrap. Scrap steel is usually contaminated with tramp elements such as copper, nickel, chromium, molybdenum, cobalt, antimony, arsenic, tungsten, tin, and zinc and some of these tramp elements remain in the finished products which can affect its quality. In addition, the nitrogen content of EAF steel is higher than BF-BOF steel. Therefore, in EAF production, if high quality control, i.e. sorting and blending of scrap is done, steels of high quality, such as stainless or engineering steel, can be produced. On the other hand, if the quality control is low then relatively low quality steels can be cost effectively produced via EAF.
Of the above product groups, rolled sections and rebar are classified as long products in which some tramp elements and a relatively high nitrogen content in steel are allowed. For flat products, groups 3, 4 and 5 above, the presence of tramp elements and gases has to be controlled, since they cause deterioration in surface quality and the mechanical properties of steel and therefore BF-BOF production is generally the preferred production route for these products.
EAF technology is however improving and, in certain countries, notably the USA which has a reliable supply of scrap, EAF technology is now being used to produce some flat products including steel plate. Part of the reason for this is the introduction of a proportion of pure iron (Direct Reduced Iron, DRI) or high quality clean scrap to the EAF to ensure that the quality of the steel is kept sufficiently high to roll flat products of the required quality.
What is the difference between closed-loop recycling and downcycling?
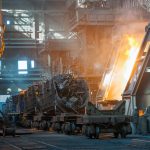
Although most UK demolition waste is now recovered following demolition (it is estimated that >90% of UK demolition arisings are currently recovered and reused in some form), it is important to know how easy it is to recover and recycle different products/materials and to understand the relative benefits of different forms of recycling and reuse.
Construction materials differ significantly in the ease with which can be recovered and recycled and if we ignore this aspect then, in effect, we are equating products that are recycled with products that are landfilled!
Metals, for example, are infinitely recyclable, i.e. they can be recycled again and again into functionally equivalent products. This is the most environmentally beneficial form of recycling and is called closed-loop recycling.
Many other construction products are downcycled into new products that are only suitable for lower grade, lower values applications because the recycled product has different, usually lower, material properties. Although waste is diverted from landfill by downcycling, only lower grade primary resources are saved. For example, crushing bricks and concrete for sub-base or general fill saves aggregates but does not save the resources required to make new bricks or new concrete.
The methodology generally used in construction to quantify these relative recycling or circular economy benefits is called Module D.
Recycling construction products is important to conserve resources and to reduce environmental impacts. Although most end-of-life building products are now recovered following demolition (it is estimated that >90% of UK demolition arisings are currently recovered and reused in some form), it is important to know how easy it is to recycle different products/materials and to understand the relative benefits of different forms of recycling and reuse.
Construction materials differ significantly in the ease with which can be recovered and recycled and if we ignore this aspect then, in effect, we are equating products that are recycled with products that are landfilled! Preserving resources through reuse and recycling is vital to achieve a more circular economy and to reduce greenhouse gas emissions.
The benefits of recycling and reuse are well understood and include:
- Reducing waste, i.e. diverting waste from landfill
- Saving resources, i.e. substituting primary production
- Saving energy and associated greenhouse gas emissions through less energy intensive reprocessing
Although these benefits apply to many commonly recycled materials, there are some important differences in material properties that influence the environmental benefit of recycling and particularly how these benefits are quantified.
Metals, for example, are infinitely recyclable, i.e. they can be recycled again and again into functionally equivalent products. This is the most environmentally beneficial form of recycling and is called closed-loop recycling. By carefully blending scrap steel it is also possible to produce new steel with superior properties and higher value, e.g. engineering and stainless steels. This is called upcycling.
Other construction products are downcycled into new products that are only suitable for lower grade, lower values applications because the recycled product has different, usually lower, material properties. Although waste is diverted from landfill by downcycling, only lower grade primary resources are saved. For example, crushing bricks and concrete for hardcore, sub-base or general fill saves aggregates but does not save the resources required to make new bricks or new concrete.
For recycling to be sustainable in the long term, it is important that the recycling process is financially viable. This is frequently the biggest hurdle to recycling, particularly for products and materials that are downcycled into lower grade, low value applications. These products often require incentives or regulation to encourage recycling.
Steel scrap, on the other hand, has a high economic value that ensures it is always highly recycled. This process is enhanced by steel’s magnetic properties that allow it to be easily recovered from mixed waste streams. An established infrastructure exists to collect, sort and process scrap efficiently.
The methodology generally used in construction to quantify these relative recycling or circular economy benefits of different products is called Module D and is explained in the next FAQ Why are UK sections produced via the primary (BF-BOF) production route?
Why are UK sections produced via the primary (BF-BOF) production route?
 production route.jpg)
Hot-rolled steel sections can be produced by either the primary BF-BOF or the secondary EAF production routes. The quality and strength of the finished product (to the same product standard) is the same irrespective of the production route.
The main reasons that the UK mainly uses the BF-BOF steel production route are historical, geographical and political. The current production split of steelmaking in the UK is 80:20 (BF-BOF:EAF). Globally, the split is 70:30.
Early steel production in the UK was located in areas with local availability of the primary raw materials iron ore and coal. However, over time, it was the proximity to deep-water ports, to enable the import of higher quality iron ore and coal/coke, that was key to the development and consolidation of primary steelmaking in the large steelworks in South Wales, Scunthorpe, Teesside and Scotland. The proximity of some steelworks to other heavy industries, such as ship-building, was also important.
The capacity of the UK’s BF-BOF steel mills, largely developed in the 1960s and 70s, greatly exceeded the declining domestic demand; which fell from a peak of 28 mt in the 1970s to just 7mt pa today. This decline has hindered the ability of UK steel producers to invest in new EAF steel mills. A contributory factor has been the high industrial electricity prices in the UK which are more than 50% above other major EU economies.
Hot-rolled steel sections can be produced via either the primary BF-BOF or the secondary EAF production routes. The quality and strength of the finished product (to the same product standard) is the same irrespective of the production route.
The main reasons that the UK mainly uses the BF-BOF steel production route are historical, geographical and political. The current production split of steelmaking in the UK is 80:20 (BF-BOF:EAF). Globally, the split is 70:30.
The UK led the Industrial Revolution in the 19th century and steel played a vital role in this. In 1875, for example, the UK accounted for nearly half of the global production of pig iron. Today the UK accounts for just 0.4% of total global steel production.
Early steel production in the UK was located in areas with local availability of the primary raw materials iron ore and coal. However, over time, it was the proximity to deep-water ports, to enable the import of higher quality iron ore and coal/coke, that was key to the development and consolidation of primary steelmaking in the large steelworks in South Wales, Scunthorpe, Teesside and Scotland. The proximity of some steelworks to other heavy industries, such as ship-building on the Clyde, was also important.
The capacity of the UK’s BF-BOF steel mills, largely developed in the 1960s and 70s, greatly exceeded the declining domestic demand; which fell from a peak of 28 mt in the 1970s to just 7mt pa today. This decline has hindered the ability to invest in new EAF steel mills and, to a large extent, also prevented major investment in upgrading the existing BF-BOF steelworks.
A contributory factor to the lack of investment in EAF steelmaking, has been the high industrial electricity prices in the UK which are more than 50% above other major EU economies, see Fig. 7.
Fig. 7. Steel electricity price disparity 2018-19, UK, Germany and France
Why is structural steel reuse not more common?
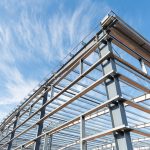
Reusing structural steel makes good environmental sense and can save money. Reuse, as opposed to the current, common practice of recycling structural steel by remelting, offers significant potential both in terms of resource efficiency and carbon emission savings.
Although technically viable, there are many practical and logistical barriers to more widespread reuse. These include the availability of reclaimed sections particularly of the desired size, volume and location, and issues relating to the quality, traceability and certification of reclaimed sections. The additional time/programme and cost of sourcing and using reclaimed steel are also barriers.
Despite the barriers, structural steel remains one of the most viable candidate products for mainstream reuse and without question, reuse will become more commonplace in the future as we embrace circular economy principles.
To help facilitate the reuse of existing structural steel, SCI has published a protocol (SCI P427) setting out recommendations for data collection, inspection, testing and design of reclaimed structural steelwork.
Reusing structural steel makes good environmental sense and can save money. Reuse, as opposed to the current, common practice of recycling structural steel by remelting, offers significant potential in terms of both resource efficiency and carbon emission savings.
Structural steel sections are inherently reusable. The process is straightforward. For example, deconstructed sections are inspected to verify their dimensional properties, tested to determine their mechanical properties and the section is then generally shot or sand-blasted to remove any coatings, and refabricated and primed to the requirements of the new project.
Despite the viability of reusing structural steel, there are many barriers to more widespread reuse. These include the availability of reclaimed sections particularly of the desired size, volume and location, and issues relating to the quality, traceability and certification of reclaimed sections. The additional time/programme and cost of using reclaimed steel are also barriers. Extra time, in general, incurs additional cost and there are many barriers centred around the increased programme associated with reclaiming and reusing structural steel including:
- The current approach of ‘just-in-time’ supply (of new steel) by stockholders and steelwork contractors
- The additional design, procurement and testing/certification time required compared to using new steel
- Increased automation in steelwork fabrication which is far less efficient when using reclaimed steel sections
- Insufficient time within new development programmes to allow for deconstruction and recovery of the steel elements rather than demolition
Despite the barriers, structural steel remains one of the most viable candidate products for mainstream reuse and without question, reuse will become more commonplace in the future as we embrace circular economy principles. To help facilitate the reuse of existing structural steel, SCI has published a protocol (SCI P427) setting out recommendations for data collection, inspection and testing to ensure that reclaimed structural steelwork can be reused with confidence.
More can also be done to facilitate reuse in the future and work is underway within the sector to support this. The proportion of recovered products that are reused will increase as design for deconstruction is better understood and a stronger market for reusable steel construction products is stimulated. The ability of the steel construction sector to reuse structural steelwork is enhanced by the standardisation of components and connections.
To help facilitate design for deconstruction and reuse, SCI has developed guidance on the design of demountable composite construction systems (SCI P428).
What is Module D and how do I use it?
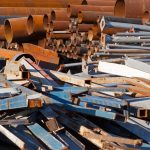
European (CEN) Standards relating to the sustainability assessment of construction works adopt a modular approach to assess and report the impacts over the life cycle of a building. These include (Module A) from the production of the construction products and their assembly into buildings, (Module B) the use of the building over its design life and (Module C) the end-of-life of the building including demolition and disposal of the demolition waste.
Module D is a supplementary module that reflects benefits and loads beyond the defined system boundary, i.e. the building lifetime, and includes reuse and recycling potentials of materials and products recovered from the end-of-life of buildings. BS EN 15804 now mandates the reporting of Module D.
Module D is calculated based on the avoided impacts of primary production. For example, if a product is recycled, the Module D benefit is the avoided impact, i.e. the impact avoided by not producing the product via the primary production route. Where there is a loss of quality, for example crushing concrete into rubble, then a value-correction factor is applied to reflect the lower value of this type of recycling.
While BS EN 15804 and BS EN 15978 make clear recommendations about how the environmental impact data should be calculated and reported, how they are used to make decisions is outside the scope of these standards.
Guidance is therefore needed to help designers and specifiers use separate values, e.g. Module A and Module D, to make decisions. Whereas reducing Module A impacts may be a current priority for many, ignoring Module D effectively equates a material that is landfilled following demolition with one that is reused or recycled. To transition to a more circular economy, we have to start incentivising design for deconstruction and reuse and set Module D targets alongside those currently being proposed for Module A.
European (CEN) Standards relating to the sustainability assessment of construction works adopt a modular approach to assess and report the impacts over the life cycle of a building project. The different life cycle stages are defined as separate information modules (A to D) to promote transparency of assessment and reporting.
The assessment boundary of a building is defined as its full life cycle, i.e. from the production of the construction products and their assembly into buildings (Module A), the use of the building over its design life (Module B) and the end-of-life of the building including demolition and disposal of the demolition waste (Module C).
Module D is a supplementary module that reflects benefits and loads beyond the defined system boundary, i.e. the building lifetime, and includes reuse and recycling potentials of materials and products recovered from the end-of-life of buildings.
It is important to note that these are ‘potentials’ that are based on things/scenarios that might happen at some point in the future, not actual impacts as is the case for Module A.
Module D is intended to be a measure of the benefits of reusable and recyclable products/materials and to encourage measures to deliver a future circular economy, for example through design for deconstruction and reuse.
Note that BS EN 15804:2019, now mandates the reporting of Modules A1-A3, C1-C4 and D for almost all construction products.
Module D is calculated based on the avoided impacts of primary production. For example, if a product is recycled, the Module D benefit is the avoided impact, i.e. the impact avoided by not producing the product via the primary production route. Where there is a loss of quality, for example crushing or downcycling concrete into rubble, then a value correction factor is applied to reflect the lower value of this type of recycling.
How is Module D calculated?
Calculation of Module D is complex and is related to some fundamental Life Cycle Assessment (LCA) concepts, including the goal and scope of assessment, etc. It is not intended to cover this complexity here.
In the context of steel however, the Module D approach can be explained relatively simply, using the following equation:
The first bracket is a measure of ‘net flow’ of the product through the building system. For example, for BF-BOF steel production with 15% scrap input and an end-of-life recovery and recycling rate of 90%, the net flow over the product life cycle is 90% – 15% = 75%.
The second bracket reflects the ‘potential saving’ achieved through recycling (or reuse) relative to primary (BF-BOF) production. For example, if we recycle a steel section we emit typically 0.5 tCO2e per tonne (by recycling) but we ‘save’ typically 2.5 tCO2e per tonne, i.e. by avoiding primary (BF-BOF) production.
For this scenario therefore Module D = (0.9 - 0.15) x (0.5 – 2.5) = -1.5 tCO2e per tonne
Note that a negative value indicates a ‘saving’ whereas a positive value indicates an additional ‘burden’.
Annex D of BS EN 15804:2019 provides end-of-life formulae for the assessment of the environmental impact of different information modules of construction products including Module D.
How to use Module D?
CEN standards, BS EN 15804 and BS EN 15978, and embodied carbon assessment guidance based on these standards, for example from RICS and the IStuctE, do not allow aggregation of any of the information Modules A to D and require Module D to be reported and communicated separately.
Despite the above, there is no definitive guidance in standards about what can and cannot be done in terms using Modules A and D to make decisions regarding building design and product selection. While BS EN 15804 and BS EN 15978 make clear recommendations about how the environmental impact data should be reported, how they are used to make decisions is outside the scope of these standards.
Guidance is therefore needed to help designers and specifiers use separate values, e.g. Module A and Module D, to make decisions. Whereas addressing or reducing Module A impacts may be a current priority for many, ignoring Module D effectively equates a material that we landfill following demolition with one that we reuse or recycle. To transition to a more circular economy, we have to start incentivising design for deconstruction and reuse and quantifying Module D benefits and setting Module D targets. Although there is uncertainty about future scenarios for reuse and recycling, particularly for long-lived products like buildings, we should not let this hinder taking action today to facilitate better utilisation of our buildings and our building products in the future.
It is recommended therefore that targets are set for Module D alongside those currently being proposed for Module A.
It is noted that some EU member states have developed national rules, assessment methods and databases of environmental impact data in which a proportion of the Module D saving can be taking into account, i.e. aggregating a proportion (typically 30-50%) of the potential Module D benefit with today’s Module A impact to derive a single value. This can help to set targets and make decision-making easier.
How to obtain BREEAM credits for responsibly sourcing structural steelwork?
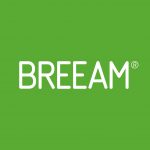
Responsible sourcing of construction products is assessed under BREEAM Mat 03 under which up to 3 credits are achievable. Recognised schemes are listed in BREEAM Guidance Note GN18.
There are situations where a product/material specified by the design team (or chosen by the constructor) is certified at manufacture but is supplied by one or more organisations that have no relevant certification. This reduces the level of assurance because the chain-of-custody/traceability is broken (so-called ‘broken chain’). This would apply where a steelwork fabricator, without responsible sourcing certification, is using steel from a certified source, i.e. steel producer.
BREEAM therefore accepts that steelwork contractors do not need to have their own responsible sourcing certification provided that they are using a recognised quality management system and they are using steel from a certified source.
Responsible sourcing of construction products is assessed under BREEAM Mat 03 under which up to 3 credits are achievable. Credits are awarded as follows:
The scope of assessment of the superstructure is defined as:
To assess a building, the assessor has to establish the BREEAM-recognised responsible sourcing certifications scheme (RSCS) certification or environmental management system (EMS) certification, if any, relevant to each product/material within the scope.
Recognised schemes are listed in BREEAM Guidance Note GN18. GN18 lists the following schemes which are currently recognised by BREEAM and their scoring.
Where the construction product has no certification, is non-compliant with the broken chain (see below) requirements or the certification type is not listed in Guidance Note 18, the score is zero.
Broken chain
There are situations where a product/material (or constituent product/material) specified by the design team (or chosen by the constructor) is certified at manufacture but is supplied by one or more organisations that have no relevant certification. This reduces the level of assurance because the chain-of-custody/traceability is broken (so-called broken chain). For example, this would apply where a steelwork fabricator, without responsible sourcing certification, is using material from a certified source.
To recognise responsible sourcing certification where it does exist in the supply chain, while reducing the risks associated with a broken chain, it is permissible to use the upstream certification score in the Mat 03 calculator where the downstream risk to responsible sourcing is considered to be low.
Specifically, it is acceptable for the following types of organisations in the supply chain (that are downstream of the organisation with certification) not to have their own responsible sourcing certification:
- Organisations that only handle or transport
- Organisations that only fabricate, assemble or install and are using a recognised quality management system to ensure the mixing and substitution of the certified upstream source with uncertified sources has not occurred
- Are operating in a jurisdiction that can demonstrate relatively robust and well enforced environmental, social and economic controls. For example:
- States which are members of the EU
- States that have declared adherence to the OECD Guidelines for Multinational Enterprises.
For the case of structural steel therefore, full benefit for responsible sourcing under BREEAM can be achieved as long as the steel producer is certified to a recognised responsible sourcing scheme (point 2 above) as long as they have a recognised quality management system in place. In the UK this is generally BES 6001 certification which the major steelwork suppliers to the UK market (British Steel, ArcelorMittal Long products Europe, Spartan UK and Tata Steel) currently have.
The full list of steel companies currently certified to BES 6001 can be seen here BRE Group: Responsible Sourcing League Tables
Further Reading
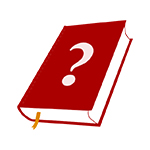
All points referenced in the frequently asked questions can be found in the detailed answer below, which provides a list of publications that information was sourced from.
- ISO 14021: Environmental labels and declarations — Self-declared environmental claims (Type II environmental labelling).
- Atherton, J., Declaration by the Metals Industry on Recycling Principles. International Journal LCA 12 (1), 2007.
- 2020 World Steel in figures. worldsteel.
- Steel Arising: Opportunities for the UK in a transforming global steel industry. University of Cambridge, 2019.
- Calculating and declaring recycled content in construction products ‘Rules of Thumb’ guide. WRAP, 2008.
- Is the desire to reduce the embodied carbon of new buildings damaging the UK steel industry? KLH Sustainability, 2019.
- Inventory of carbon and energy (ICE) V2.0 Annex B: Methodologies for recycling, 2011.
- The recycling and reuse survey https://www.steelconstruction.info/The_recycling_and_reuse_survey
- Moynihan, M. C., Allwood, J. M. Utilization of structural steel in buildings, Proc. R. Soc. A 470 (2168) (2014) 20140170.
- Dunant, C., Drewniok, M., Eleftheriadis, S., Cullen, J., Allwood, J. Regularity and optimisation practice in steel structural frames in real design cases. Resour Conserv Recycling 2018;134:294–302.
- Orr, J., Drewniok, M., Copping, A., Emmitt, S., Ibell, T. Minimizing energy in construction-survey of structural engineering practice. Cambridge: MEICON project Report; 2018. 12.
- Drewniok, M., Campbell, J., Orr, J. SI for the paper: The Lightest Beam Method – a methodology to find ultimate steel savings and reduce embodied carbon in steel framed buildings [Dataset]. https://doi.org/10.17863/CAM.47336, 2020.